The explosion in human population is a problem to the survival of human existence, so there is an urgent need to increase the production capacities of products, including vaccines, therapeutic molecules, antibodies, industrial enzymes, developing plant oil as well as biofuels and produce them at low-costs. Plant biotechnology based production was considered to be a solution for such a major global challenge. However, this approach is not yet exploited for the production of any recombinant protein for any major application so far. This was mainly due to lack substrates and/or high level expression of enzyme activity in a desired plant tissue making the whole process uneconomical. So, a combine of many molecular biology techniques, such as seeking synthetic or modified genes and use most sensible genetic engineering strategy can solve above problem. In this overview, I would like to focus just some topics which I had worked, and hope they may be support new products or increasing a product and produce at low-cost.
1. Boost astaxanthin and EPA in soybean and camelina seeds
 Astaxanthin accumulation turn soybean seed to orange (right) compare with non-transgenic seeds( left)
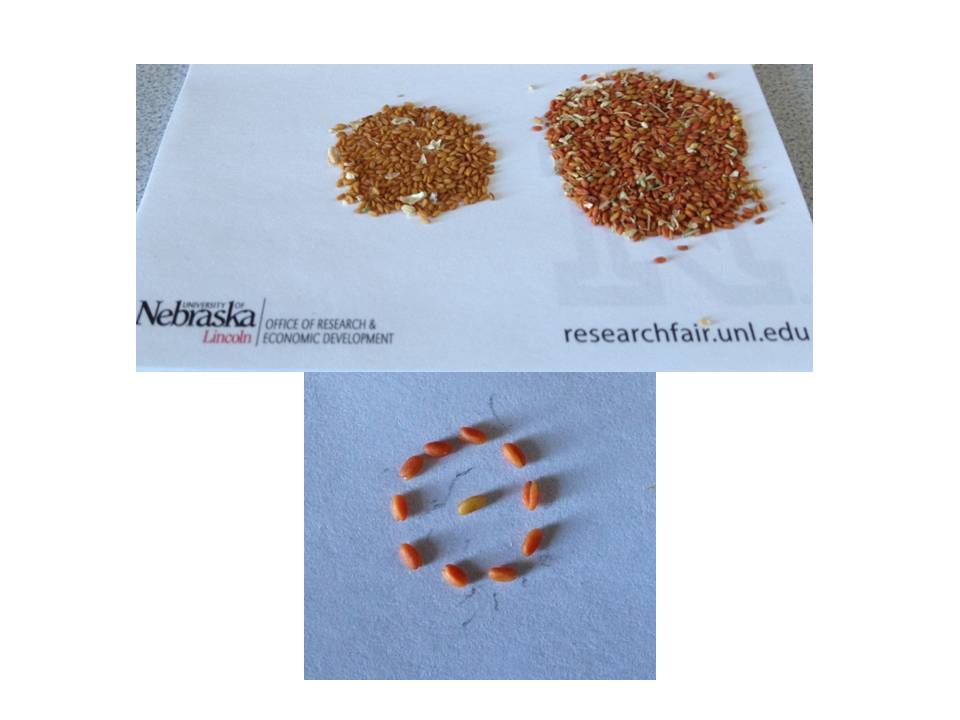
Camelina seeds look dark orange (top right and circle-bottom) compare with wild type seed (top left or center-bottom)
2. Redirection of Metabolic Flux for High Levels of Omega-7 Monounsaturated Fatty Acid Accumulation in Camelina seed
Seed oils enriched in omega-7 monounsaturated fatty acids, including palmitoleic acid (16:1D9) and cis-vaccenic acid (18:1D11), have nutraceutical and industrial value for polyethylene production and biofuels. Existing oilseed crops accumulate only small amounts (<2%) of these novel fatty acids in their seed oils. We demonstrate a strategy for enhanced production of omega-7 monounsaturated fatty acids in camelina (Camelina sativa) and soybean (Glycine max) that is dependent on redirection of metabolic flux from the typical delta 9 desaturation of stearoyl (18:0)-acyl carrier protein (ACP) to delta 9 desaturation of palmitoyl (16:0)-acyl carrier protein (ACP) and –coenzyme A (CoA). This was achieved by seed-specific co-expression of a mutant delta 9-acyl-ACP and an acyl-CoA desaturase with high specificity for 16:0-ACP and -CoA substrates, respectively. This strategy was most effective in camelina where seed oils with ~17% omega-7 monounsaturated fatty acids were obtained. Further increases in omega-7 monounsaturated fatty acid accumulation to ~ 65% of the total fatty acids in camelina seeds were achieved by inclusion of seed-specific suppression of 3-keto-acyl-ACP synthase II and the FatB 16:0-ACP thioesterase genes to increase substrate pool sizes of 16:0-ACP for the delta 9-acyl-ACP desaturase and by blocking C18 fatty acid elongation. Seeds from these lines also had total saturated fatty acids reduced to ~5% of the seed oil versus ~12% in seeds of non-transformed plants. Consistent with accumulation of triacylglycerol species with shorter fatty acid chain-lengths and increased monounsaturation, seed oils from engineered lines had marked shifts in thermotropic properties that may be of value for biofuel applications.
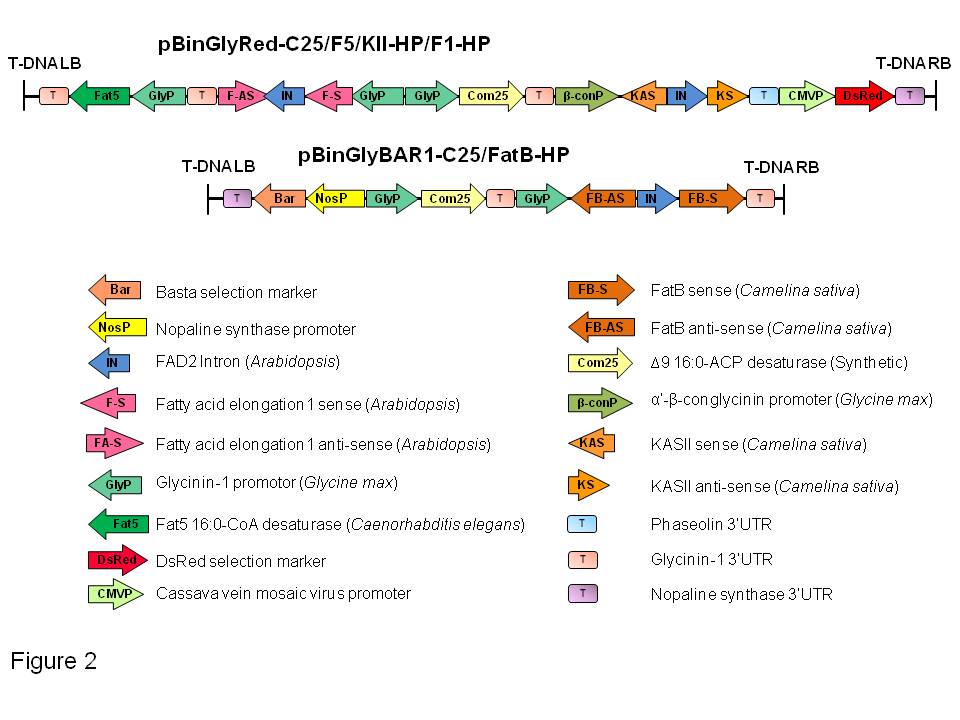

3. Camelina Seed Transcriptome: A Tool for Meal and Oil Improvement and Translational Research
Camelina (Camelina sativa), a Brassicaceae oilseed, has received intense interest as a biofuel crop and production platform for industrial oils. Limiting wider production of camelina for these uses is the need to improve the quality and content of the seed protein rich-meal and oil, which is enriched in oxidatively unstable polyunsaturated fatty acids that are deleterious for biodiesel. To identify candidate genes for meal and oil quality improvement, we built a transcriptome reference from 2,047 Sanger ESTs and over 2 million 454-derived sequence reads, representing genes expressed in developing camelina seeds. The transcriptome of ~60K transcripts from 22,597 putative genes includes camelina homologs of nearly all known seed-expressed genes, suggesting a high level of completeness and usefulness of the reference. These sequences included candidates for 12S (cruciferins) and 2S (napins) seed storage proteins (SSPs) and nearly all known lipid genes, which have been compiled into an accessible database. To demonstrate the utility of the transcriptome for seed quality modification, seed-specific RNAi lines deficient in napins were generated by targeting 2S SSP genes, and high oleic acid oil lines were obtained by targeting fatty acid desaturase 2 (FAD2) and fatty acid elongase 1 (FAE1). The high sequence identity between Arabidopsis and camelina genes was also exploited to engineer high oleic lines by RNAi with Arabidopsis FAD2 and FAE1 sequences. It is expected that this transcriptomic data will be useful for breeding and engineering of additional camelina seed traits and for translating findings from the model Arabidopsis thaliana to an oilseed crop.
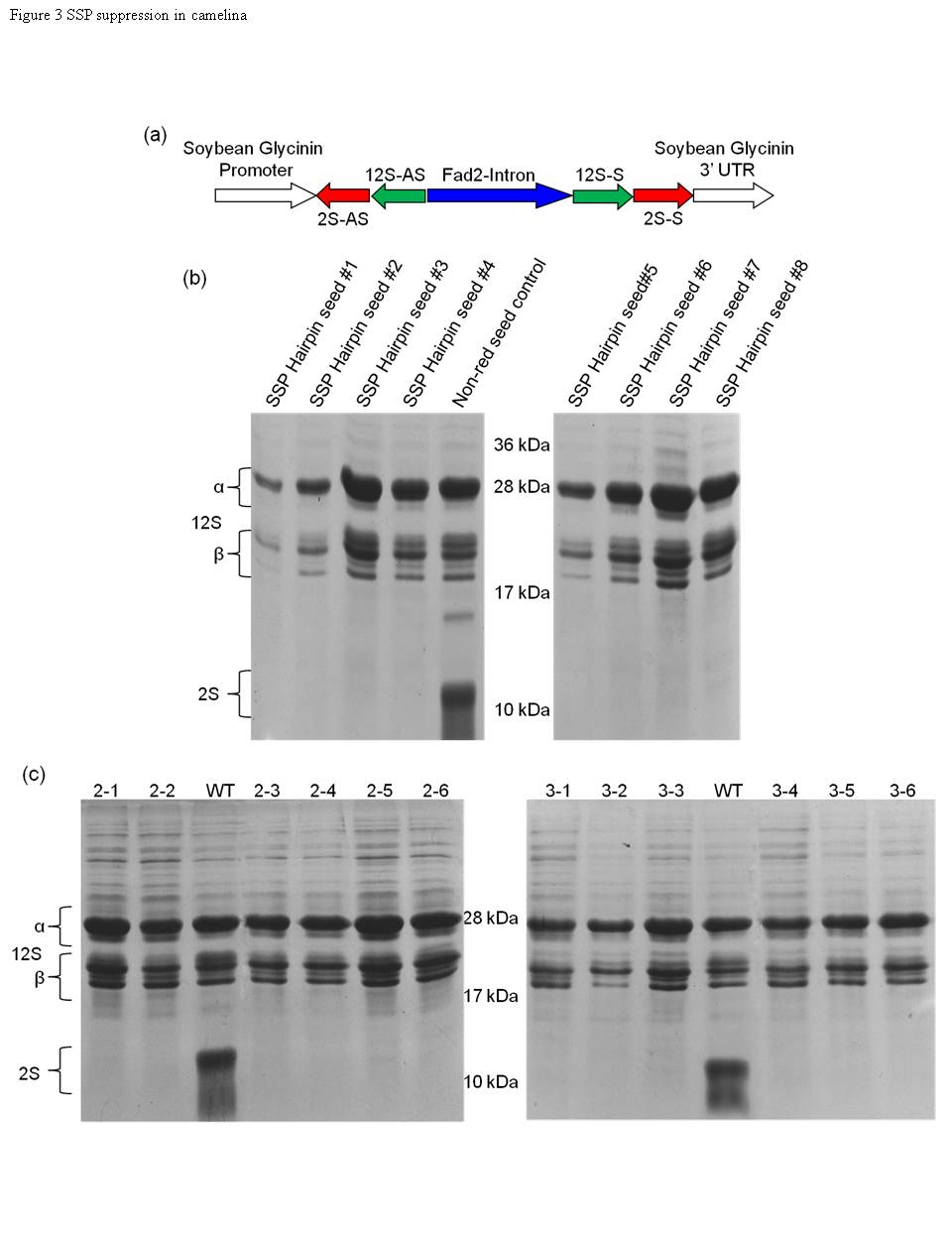
4. Omega7, turn nothing into 70%
The essential unsaturated omega-7 fatty acid which include palmitoleic acid and cis-vaccenic acid are of particular importance, because it confers fluidity to the membrane, whilst having a low susceptibility to oxidation. Lipid peroxidation by free radicals results in the disintegration of membranes and a resultant loss of function, leading to ulceration of the digestive tract, a disturbed epidermal barrier in the skin, and dryness of the genital tract lining. Omega-7, together with the other classes of omega-7 play an important role in regulating immune function and the inflammatory response. Thus omega-7 fatty acids promote tissue regeneration and anti-inflammatory action in the skin and mucosa. http://www.plantphysiol.org/content/154/4/1897.full.pdf
Gas chromatograph-mass spectrometer (GCMS) analysis fatty acid single seed of control (top) and transgenic (bottom) seeds
5. Engineering Plant Oils
Using genetic manipulation to modify the activity of a plant enzyme, we have converted an unsaturated oil in the seeds of a temperate plant to the more saturated kind usually found in tropical plants. The research had published by the Proceedings of the National Academy of Sciences (PNAS) with title “Modulating seed Beta-ketoacyl-acyl carrier protein synthase II level converts the composition of a temperate seed oil to that of a palm-like tropical oil”, vol.104, 2007.
While conversion of an unsaturated oil to an oil with increased saturated fatty acid levels may not sound like a boon to those conscious about consuming unsaturated fats, the development of new plant seed oils has several potential biotechnological applications, for one thing, the new tropical-like oil has properties more like margarine than do temperate oils, but without the trans fatty acids commonly found in margarine products. Furthermore, such engineered oils could be used to produce feedstocks for industrial processes in place of those currently obtained from petrochemicals. We also suggest that the genetic manipulation could work in the reverse to allow scientists to engineer more heart-healthy food oils.
Scientists have known for a long time that the ratio of saturated to unsaturated fatty acids plays a key role in plants’ ability to adapt to different climates, but to change this ratio specifically in seed oils without changing the climate is an interesting challenge, we sought to gain a better understanding of the enzymes and metabolic pathways that produce these oils to find ways to manipulate the accumulation of fats using genetic techniques.
We focused on an enzyme known as KASII that normally elongates fatty acid chains by adding two carbon atoms. The longer 18-carbon chains are more likely to be acted on by enzymes that desaturate the fat. So the scientists hypothesized that if they could prevent the chain lengthening by reducing the levels of KASII, they could decrease the likelihood of desaturation and increase the level of saturated fats in the plant seeds.
The hypothesis was supported by the fact that scientists had previously identified a plant with a mutated KASII that showed reduced enzyme activity, and these plants were able to accumulate more saturated fats than was normal. We set out to reduce KASII activity with the use of RNA-interference (RNAi) to see if they could further increase the level of saturation in plant seed oils.

Gas chromatograph-mass spectrometer (GCMS) analysis fatty acid single seed of KasII-Hairpin Antisense seed
Suppression of kasII enzyme activity by KasII Hairpin Antisense due to reducing 18:0, 18:1 (delta 9), 18:2 and 18:3 fatty acid (diagram at very top) and result analysis fatty acid by GCMS of transgenic (bottom) and control (top) seeds showed that an accumulation of 16:0 Fatty acid due to enhance 16:1 delta 9 and 18:1 delta11 fatty acids (Source: unpublished)
We performed experiments on Arabidopsis, a plant commonly used in research. Like other plants from temperate climates (e.g., canola, soybean, and sunflower), Arabidopsis contains predominantly 18-carbon unsaturated fatty acids in its seed oil. Tropical plants, in contrast (e.g. palm), contain higher proportions (approximately 50 percent) of 16-carbon saturated fatty acids.
The results were surprising. The genetic manipulations that reduced KASII activity resulted in a seven-fold increase in 16-carbon unsaturated fatty acids up to an unprecedented 53 percent in the temperate Arabidopsis plant seed oils. These results demonstrate that manipulation of a single enzyme’s activity is sufficient to convert the seed oil composition of Arabidopsis from that of a typical temperate pant to that of a tropical palm-like oil. It is fascinating and potentially very useful to know that we can change the oil composition so drastically by simple specific changes in seed oil metabolism, and that this process can occur independently from the adaptation to either tropical or temperate climates.
For example, such a technique could lead to the engineering of temperate crop plants to produce seeds containing saturated oils that can be further modified to produce renewable feedstocks for industrial processes. Such renewable resources could help reduced dependence on petroleum.
Conversely, methods to increase the activity of KASII, and therefore the production of 18-carbon desaturated plant oils, may provide a useful strategy to limit the accumulation of saturated fatty acids in edible oils, leading to more healthful nutrition.
6. RNAi Gene Suppression Approach
Antisense (AS) and hairpin (HP) RNA interference (RNAi) targeted gene suppression technologies have been used to modify seed oil composition. Larger numbers of AS transgenics have to be screened to achieve a targeted level of suppression compared to RNAi. We hypothesized combining AS with RNAi might result in enhanced gene suppression compared to either method individually. AS and HP-RNAi were combined as hairpin-antisense (HPAS) constructs containing ~125bp sense and antisense portions of an untranslated region of the target gene separated by an intron containing an antisense copy of a portion of the target coding region. The ?12-desaturase FAD2, the ?3-desaturase FAD3 and ?-ketoacyl-ACP synthase (KAS) II were targeted in Arabidopsis to evaluate changes in oil composition with AS, HP and HPAS constructs driven by the phaseolin promoter. Modest but statistically significant enhancements in oilseed phenotypes were observed with HPAS relative to AS and HP-RNAi.
 Phenotypes for HPAS suppression of FAD2 and FAD3 were indistinguishable from their strongest mutant alleles. Our data suggest that HPAS may be useful for: 1) Achieving levels of suppression comparable to those of gene knockouts in a tissue specific manner. 2) Maximizing suppression of suboptimal RNAi constructs and 3) Minimizing the screening of transgenics to achieve desired oilseed composition. The research had published online by Journal of American Oil Chemist Society, with title “Altering Arabidopsis Oilseed Composition by a Combined Antisense-Hairpin RNAi Gene Suppression Approach” volume 86, 2009 pp. 41-49.
7. T7RNA polymerase system for overexpression in plant
Use of the T7 expression system (based on T7 RNA polymerase) to overproduceforeign proteins in microbial systems has revolutionized the recombinant protein production. And therefore, T7 expression system is the most reliable and common method to produce recombinant proteins for routine lab use as well as by the pharmaceutical and other industries. So far the T7 system has met with very little success in animal systems.
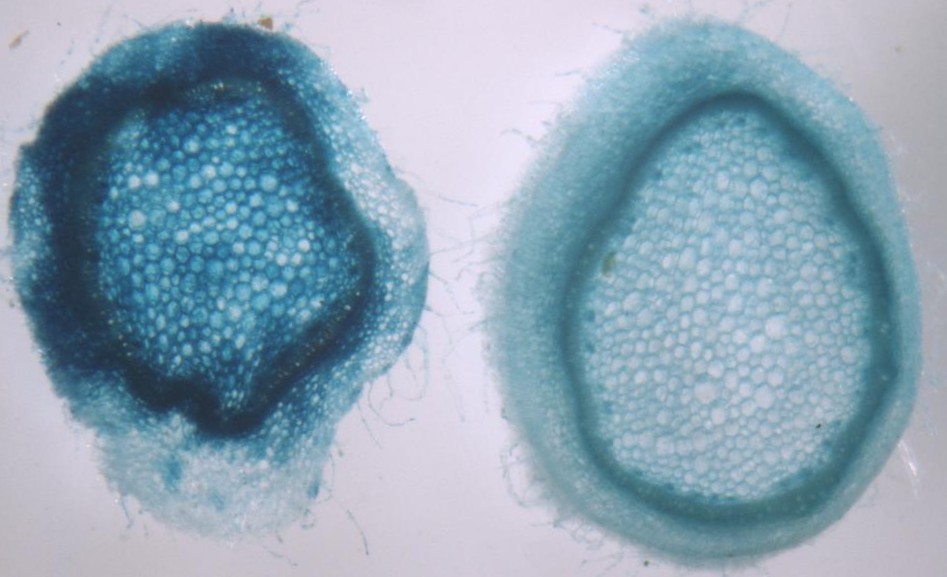
Cross section of stems show that a high GUS gene expression at T7RNAP system (left) than a regular system (right)
We are reporting the development of a new powerful tool for plant expression system based on the T7 RNA polymerase that may have major impact on plant biotechnology and on functional genomic studies as well. Our results not only demonstrate the use of T7 system for simple overexpression of foreign gene in higher plants but also in a highly tissue specific manner. Also, in these studies we demonstrate that the T7 system can be used to regulate the expression of foreign gene through inducible mechanisms, akin to inducible expression of recombinant proteins E. coli based on T7 system. The tissue specific and inducible high level expression was found to work in both dicot (tobacco) and monocot (rice) plants. As our approach is quite novel and the expression levels obtained are exceptionally very high with wide application in both academic research and in industry. This research had patented (http://india.bigpatents.org/view/37991/258ecf14252) and published in Journal of Plant Biotechnology, with title “Bacteriophage T7 RNA polymerase-directed, inducible and tissue-specific over-expression of foreign genes in transgenic plants”, volume 2, Issue 4, 2004. Pages: 301–310.
In addition, I designed a lot of binary vectors for plant transformation, used a lot of genes such as KasII, Fad2, Fad3, FatB, Com25, T7RNAP, GusA, Ds-Red, Zs-green, Zs-yellow, GFP, Ferritin, Vitamin A, GluB1, HBsAg etc. Beside above technology, many promoters such as Phaseolin, 35S CaMV, rbcS-3A, kin1, cor6.6, gluB1, P170, T7 etc have been used for expression at different plant tissues specific in different purposes. And I have expert at almost molecular biology techniques.
Tam Nguyen, Ph.D.
www.omega-7.org |